VandyGRAF Initiative
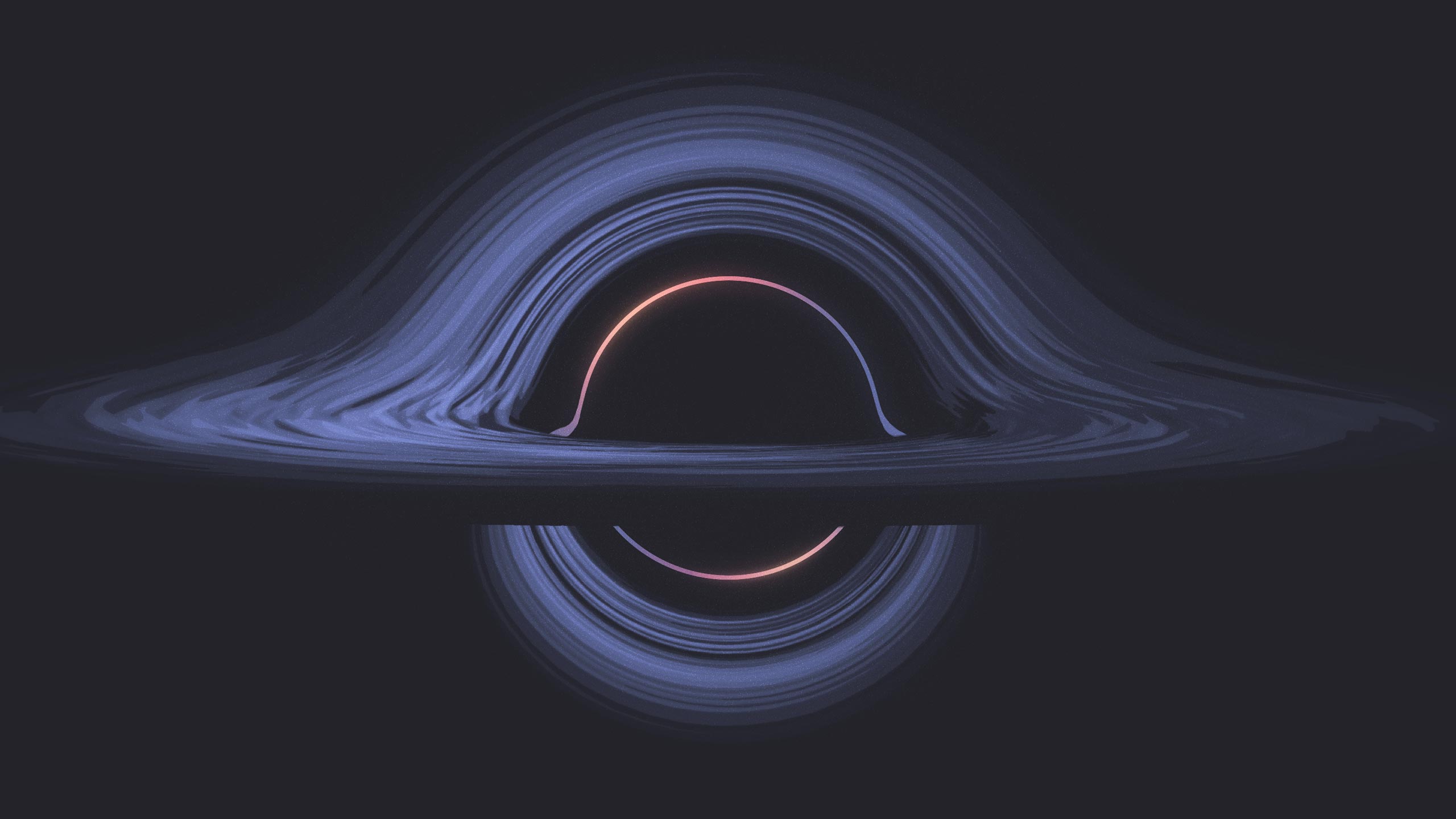
The Vanderbilt Initiative for Gravity, Waves, and Fluids is an interdisciplinary research venture providing mathematicians, physicists, and astrophysicists with the resources and space to connect and collaboratively work on problems of outstanding scientific merit, such as:
- General relativity: theoretical, mathematical, numerical, or experimental, including, but not restricted to, black holes, gravitational radiation, and multimessenger astrophysics.
- Fluid mechanics: theoretical, mathematical, numerical, or experimental, including, but not restricted to, relativistic fluids far from equilibrium.
- Evolution of partial differential equations related to fluids and gravity, including, but not restricted to, the geometric analysis of waves and fluids.
- The physics and mathematics of neutron star mergers and high-energy nuclear collisions.
VandyGRAF Seminar Series
All VandyGRAF talks will take place in the Chapel in the 17th&Horton building, unless indicated below.
Khwahish Kushwah, Universidade Federal Fluminense
December 6, 12:00 pm - 1:30 pm